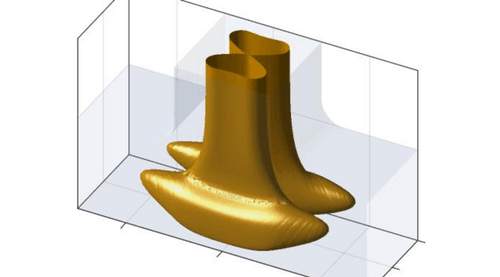
Particles carried by a fluid moving through a T-shaped pipe junction can be trapped, even though the fluid passes unhindered through the intersection. Now researchers have found through simulations of particle trajectories that this phenomenon occurs in a surprisingly large volume of the junction. For particles approaching the junction, as much as 25 percent of the cross section of the input pipe can lead them to become trapped. The results could help researchers studying a wide range of problems, such as diseases related to deposits in arteries and malfunctions in industrial fluid distribution networks.
Four years ago, Howard Stone, of Princeton University in New Jersey, and his colleagues reported their discovery that low-density particles in a fluid moving through a T-shaped intersection could accumulate at the junction, despite the steady flow of the fluid. The result was surprising and could be important for any branching pipe network because the trapping occurs over a wide range of branch angles and flow speeds.
Stone and his colleagues then showed that the effect is likely linked to a process called vortex breakdown: A complicated pattern of eddies forms as a result of the sharp turns in the pipe, and above a certain flow speed, some fluid moves back against the main flow in each of the two branches. This reversed flow produces small regions where the flow speed is zero.
The researchers could reproduce this flow pattern with numerical modeling, but the flow trajectories followed by a fluid are not necessarily the same as the paths of the particles it carries. Particles such as the hollow glass beads used in the experiments have inertia and experience extra forces, especially drag. In addition, simulations showed that vortex breakdown occurs in one or two “bubbles” in each output branch, and it wasn’t clear what would cause particles to cross into these bubbles and become trapped.
To fully explain how trapping occurs, the Princeton team joined forces with an applied mathematics group headed by George Haller of the Swiss Federal Institute of Technology (ETH) in Zurich. Haller’s group simulated the individual particle paths through the T-junction and then applied the concept of Lagrangian Coherent Structures, a technique that can reveal large-scale particle flow structures amongst myriad particle trajectories. The result was startling: Trapping did not just happen for particles that came close to the zero-velocity locations. There were sizable regions surrounding the vortex breakdown zones from which particles could not escape. These regions, which often came in pairs, were shaped like the anchor of a ship, with a curved bottom that spread into the two outlets of the junction. Under some conditions, the regions were wide enough to touch the walls of the pipe.
The simulations confirmed the observations of particle accumulation points. Once drawn into the anchor-shaped regions, particles spiraled into specific points where they settled indefinitely. “These anchors are domains of attraction; if you’re in them, you’re doomed,” says Haller.
The results show that the anchor-shaped structures are stable for a range of junction angles, flow speeds, and particle densities, consistent with the Princeton team’s earlier experiments. Both teams were surprised that from the point-of-view of a particle approaching the junction, up to 25% of the cross-sectional area of the input pipe ultimately leads to trapping, according to the simulations of particle trajectories. The researchers also predicted that trapped particles could remain trapped despite interactions with other particles, and they found confirmation by digging up video from old experiments. As expected, a particle nudged from its stationary location by another particle passing close to the trapping region would soon spiral back to its original resting place.
One of the conditions for trapping is that the particles must be less dense than the fluid. Stone points out that bubbles in an industrial flow network such as a heat exchanger could accumulate to form a large buildup of gas. However, the new results could help engineers tweak their designs to prevent this. A small disturbance to the flow, such as a piece of wire placed across the pipe before the junction, could change the flow enough to stop the anchor regions from forming.
The fact that the anchor regions are so much larger than the regions of vortex breakdown is the biggest surprise to Amy Chen, a fluid dynamics researcher at Okinawa Institute of Science and Technology in Japan. She suggests that there will be a range of ways to harness the effect for the biotech industry, for trapping, studying, and separating particles such as cells.