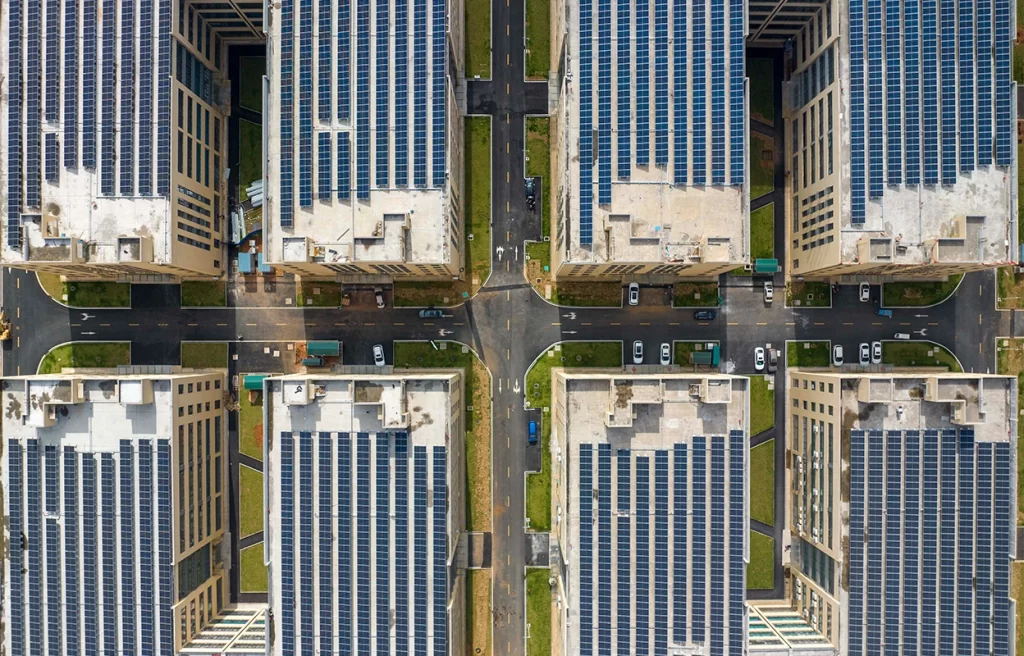
On the outskirts of Brandenburg an der Havel, Germany, nestled among car dealerships and hardware shops, sits a two-storey factory stuffed with solar-power secrets. It’s here where UK firm Oxford PV is producing commercial solar cells using perovskites: cheap, abundant photovoltaic (PV) materials that some have hailed as the future of green energy. Surrounded by unkempt grass and a weed-strewn car park, the factory is a modest cradle for such a potentially transformative technology, but the firm’s chief technology officer Chris Case is clearly in love with the place. “This is the culmination of my dreams,” he says.
The firm is one of more than a dozen companies betting that perovskites are finally poised to push the global transition to renewable energy into overdrive. A few niche perovskite-based PV products are already on the market, but announcements this year signal that many more are set to join them. Case says that end users should get their hands on solar panels made from Oxford PV’s cells around the middle of next year, for example. In May, a large silicon PV manufacturer, Hanwha Qcells, headquartered in Seoul, said it plans to invest US$100 million in a pilot production line that could be operational by the end of 2024.
Silicon is the workhorse material inside 95% of solar panels. Rather than replace it, Oxford PV, Qcells and others are piggybacking on it — layering perovskite on silicon to create so-called tandem cells. Because each material absorbs energy from different wavelengths of sunlight, tandems could potentially deliver at least 20% more power than a silicon cell alone; some scientists project much greater gains.
Perovskite supporters say that this extra electricity could more than offset the additional costs of tandem cells, particularly in crowded urban areas or industrial sites where space is at a premium. “Our biggest initial demand is from utilities, because they simply don’t have enough accessible land,” says Case.
As perovskite–silicon tandems get closer to market, excitement has boiled over into headlines predicting that a “revolutionary” “miracle material” is “about to change the world”. The reality is that the industry faces at least two major challenges in its battle to transform the solar market.
First, published research shows that the perovskites’ performance declines much more quickly than silicon when they are exposed to moisture, heat and even light. Oxford PV says it has done private research that’s overcome this issue. But “for commercial manufacturing, I would say stability is the key challenge that still remains”, says Fabian Fertig, Qcells’ director of research and development for wafers and cells, who leads the company’s development of perovskite–silicon tandems.
And second, some analysts see perovskites — at least in the short term — as largely irrelevant to the growth of solar power. Silicon modules have become incredibly cheap and efficient over the past decade, and companies in China continue to expand manufacturing capacity at a startling rate. In 2022, the world had about 1.2 terawatts (TW) of generating capacity from solar power, which in turn provided around 5% of global electricity generation. Energy strategists suggest that the world will need 75 TW by 2050 to meet climate goals. This requires installations to rise above 3 TW per year by the mid-20301, but the silicon PV industry is projected to achieve that, making it one of the rare green-technology areas that is on track (see ‘Solar’s expansion plans’).
“The technology we have is definitely good enough to generate as much solar electricity as we can use around the world,” says Jenny Chase, a solar analyst at the consultancy BloombergNEF who is based in Zurich, Switzerland.
So perovskites are about to face their biggest test: a meeting with the brutal economics of the notoriously competitive PV market.
Broken records
The enthusiasm for perovskites has been sustained by remarkable improvements in their performance, achieved by tweaking the composition of both the crystals and the solar cells made from them. (The term ‘perovskite’ describes the crystal structure of a naturally occurring mineral; the perovskites used in solar cells are synthetic crystals that mimic this structure, but can be made of many materials.)
In 2009, a cell made from a simple perovskite called methylammonium lead iodide converted just 3.8% of sunlight’s energy into electricity. Now, the efficiency record from a cell made solely from perovskite materials stands at 26.1%. That’s just a fraction of a percentage point less than the champion silicon cell (see ‘Perovskite efficiency gains’). What’s more, perovskite cells require very thin light-absorbing layers, and the materials involved are typically low cost and abundant. That leads advocates to argue that if perovskite cells were produced on the scale of silicon cells, they would have a lower energy and material footprint.
These record-efficiency comparisons don’t reflect commercial reality, however. The best perovskite cells made in labs are typically smaller than a postage stamp — the current leader is closer to the size of a sesame seed — and they might operate for only a few days or weeks before their performance degrades. They’re often made by dropping solutions of materials on to a spinning plate in a process called spin coating, which is impractical for large-scale manufacturing. “Most of the reports of very high efficiency have components in there that are clearly going to lead to instability,” says Henry Snaith at the University of Oxford, UK, who is a co-founder and chief scientific officer at Oxford PV. The practicalities of manufacturing large cells and integrating them into solar panels further curb real-world efficiency.
The non-tandem perovskite cells that have made it to market offer relatively low efficiency and short lifetimes. Saule Technologies, based in Warsaw, produces flexible perovskite cells that power small electronic price tags or serve as energy-harvesting sunblinds, offering 10% efficiency in full sunlight and lifetimes of “several years”. Microquanta in Hangzhou, China, has delivered enough perovskite solar panels to generate 5 megawatts (MW) of electrical power for its customers, including a local fish farm. Their efficiencies are around 13%, and their performance degrades twice as quickly as a silicon module. “At this moment, I have to admit that perovskite is not yet as stable as silicon,” says BuYi Yan, the company’s co-founder and chief technology officer.
Commercial silicon cells are typically larger than an A5 sheet of paper, and these are assembled into 2-metre-long modules — the building blocks of larger panels and arrays — that have an efficiency of around 22–24%. The modules typically come with a warranty that guarantees at least 80% of their original performance after 25 years — that is, a loss of less than 1% efficiency per year.
Most of the cells and almost all of the silicon wafers that make up these products are made in China, where economies of scale and technological improvements have cut the cost of a solar panel by about 90% since perovskites made their debut in 2009 (see ‘Solar power is incredibly cheap’). Indeed, it now costs more to install a silicon panel and connect it to the electricity grid than it does to make it in the first place2. The typical cost of generating electricity over the lifetime of a silicon solar array is now as low as US$0.03–0.06 per kilowatt hour, making it the cheapest source of electricity in most sunny countries, says Chase.
Compared with this silicon success story, the perovskite products are hardly likely to upend the solar power market, Chase says. “No one wants a solar module that doesn’t last 25 years. They’re just not worth it.”
Tandem tech
But perovskite proponents say that tandem cells are superior to silicon in other ways. Silicon cells are nearing peak performance: theory predicts that their efficiency cannot rise much above 29%, with practical modules likely to max out at around 24–27%3. “We’re reaching the limit of what we can do reasonably with silicon,” says Tonio Buonassisi, a PV researcher at the Massachusetts Institute of Technology (MIT) in Cambridge. Yet adding a perovskite cell produces a theoretical maximum efficiency of roughly 45%. “It’s offering the potential to get 25–50% more power out of the panels. I think that’s an exciting vision,” says perovskite researcher Michael McGehee at the University of Colorado, Boulder.
In a typical tandem device, the perovskite cell is positioned above the silicon cell; each cell is made of multiple layers that all play a part in turning light into electricity (see ‘Anatomy of a tandem solar cell’). Sunlight hits the perovskite first and releases electrons from the material, leaving behind positively charged ‘holes’. The electrons travel into an adjacent charge-collection layer, and onwards to an electrode; holes migrate in the opposite direction. A similar process happens in the silicon cell, which is better at absorbing the lower-energy photons missed by the perovskite cell. Fiddling with the chemistry and nanoscale engineering of the perovskite cell has delivered performance improvements that have quickly translated to better tandem cells. In early November, Chinese solar behemoth LONGi, headquartered in Xi’an, announced it had made a tandem cell measuring 1 square centimetre that has an independently verified record efficiency of 33.9%.
Manufacturers haven’t yet demonstrated this kind of efficiency for commercial-scale tandem cells, but in May Oxford PV announced the highest-performing perovskite–silicon tandem cell to roll off a production line, with 28.6% efficiency. Although the device was slightly smaller than typical silicon cells, the company’s Brandenburg factory is now making larger tandem cells that are being assembled into full-sized modules offering about 24% efficiency, a number that continues to rise.
Frugal approach
Oxford PV’s manufacturing process starts with silicon wafers that are mostly imported from China. The wafers pass through a series of chambers resembling conjoined refrigerators. Inside, clouds of ions build up layers of the cell in a process called physical vapour deposition. It’s slower than solution-based methods, but produces extremely high-quality films. A similar process creates the perovskite cell.
The company bought its Brandenburg factory from German manufacturer Bosch in 2016, complete with equipment for solar-cell production. “Initially, virtually everything we got at auction or used,” Case says with evident delight. His frugal approach to solar innovation dates back to the 1980s — as an enthusiastic 20-something, he bought stacks of discounted PV cells to build modules for his own ‘solar house’. This do-it-yourself approach shines through at the factory: Case had specially-tooled frames made so that Oxford PV’s cells would be compatible with Bosch’s legacy equipment, for example.
The finished tandems are delivered to Oxford PV’s customers: mostly European solar-panel manufacturers, who assemble the cells into larger modules. For now, those manufacturers are still conducting their own tests on the modules. It could be late 2024 before any devices are installed for end users, including a large construction firm and an energy company that have already ordered modules.
Working at full tilt, the plant could produce up to 50 MW of cells per year — roughly 5 million of them. But it’s a minnow compared with the vast silicon solar plants in China, which produce gigawatts (GW) of cells each year. And because of its small-scale operation, Oxford PV’s tandems cost twice as much to manufacture as a silicon cell does.
But if Oxford PV can also achieve gigawatt-scale production, that price difference would come down dramatically, says Case, which should bring the cost of generating electricity from tandem cells below that from silicon modules.
Stability challenge
About 90 minutes’ drive south from the Brandenburg factory, in Thalheim, one of Oxford PV’s competitors is testing its own prototype tandems. Before European subsidies began to dry up and Asian companies grew to dominate the solar market, Thalheim and its surrounding areas were the heartland of PV manufacturing, also known as Solar Valley. Here, Qcells has an enormous PV research and development facility. There are no unkempt lawns at Qcells — instead, small robot mowers trundle around the grounds, keeping the grass neatly trimmed before returning to their solar-powered garages.
Inside one of the site’s vast buildings, Qcells conducts ‘accelerated ageing’ tests to check that its silicon modules are robust — including sealing modules inside humid, sauna-like conditions for about four months, exposing them to bright simulated sunlight and bombarding them with artificial hailstones. (Although these tests are industry-standard, Qcells prides itself on using particularly extreme conditions.) It conducts similar tests on the tandem cells, and the results feed into predictions of how power output will decline over years of real-world use.
The details of stability tests could make or break perovskite tandems. Perovskite efficiency records grab the headlines, but only in the past few years have researchers started to make significant progress on their longevity. “It’s really the stability that is the bottleneck,” says Heping Shen, a perovskite researcher at the Australian National University in Canberra.
Although perovskites have a nasty habit of decomposing on contact with air or water, this can be prevented by encapsulating tandem cells in an impermeable coating, a common tactic in the solar industry. But, unlike silicon, perovskites also have in-built degradation mechanisms that encapsulation cannot solve. Some of the perovskite’s ions can move around during operation, for example, or escape into adjacent layers. This can create defects that enable electrons and holes to recombine before they can be turned into electricity, wasting the energy that created them. Light and heat tend to exacerbate these degradation mechanisms.
To keep the perovskites’ ions in the right places, researchers have fine-tuned their composition and added nanometre-thick protective layers to the cells. Proving that these measures are effective is a key task for the European Union’s €19-million (US$20.7-million) PEPPERONI project, an academic–industry partnership launched in November 2022 that includes Qcells. The four-year project aims to develop tandem modules with 26% efficiency that operate reliably for more than 30 years and are suitable for mass manufacturing. If these can be produced on a large scale, Fertig says that they should be able to generate electricity at a typical cost of €0.025 per kilowatt hour — competitive with silicon PV.
“The materials, the ingredients, the processes, everything is going in the direction that the technology can be scaled to a very large volume in the near future,” says Steve Albrecht, a perovskite researcher at the Technical University of Berlin. He also leads a team at the Helmholtz Centre for Materials and Energy in Berlin that is involved in PEPPERONI.
Although accelerated-ageing tests are offering promising results, the truest test for a tandem module is to simply install it outdoors and to monitor its performance for years. So far, results from only a few outdoor studies have been published, and most of these are from academic groups rather than the companies that are commercializing the tandems. “There’s simply not a lot of data out there,” says Stefaan De Wolf, a PV researcher at King Abdullah University of Science and Technology (KAUST) in Saudi Arabia, whose team reported in February on the rapid degradation of a tandem cell in the country’s hot and humid conditions4.
Qcells is not revealing details about its outdoor tests, although it has performed some at its Thalheim facility. As for Oxford PV, Case says the company has done accelerated-ageing tests and a couple of years of outdoor testing. Together, these tests — the results of which are unpublished — show that the best tandem cells lose only about 1% efficiency in their first year of operation, with a smaller rate of decline thereafter, he says. For now, he’s telling customers that the tandems should have a similar operational lifetime to a conventional silicon module.
Although there have been concerns about the presence of toxic lead in perovskites, a growing number of studies suggest that it poses little threat in a well-encapsulated panel5. Others note that a standard silicon panel can have ten times more lead in its soldered contacts than an entire perovskite panel does. Still, most companies plan take-back programmes to manage and recycle panels that have reached the ends of their lives.
A lot of ifs
Chase agrees that if the perovskite companies’ hopes are realized, the greater power density offered by tandems could prove popular among consumers. “If you could do that; and it was stable; and it didn’t cost too much in terms of manufacturing equipment or processes; then that would be quite exciting, because you’d probably get a lower-cost, higher-value module. But there’s a lot of ifs in that.”
The perovskiteers are confident they can turn those ‘ifs’ into reality. They add that homeowners are desperate to squeeze more power from rooftop panels, and might pay a premium for tandems that can feed heat pumps, electric vehicles and other accoutrements in their increasingly electrified homes. “Rooftop areas tend to be too small now for what the household needs. So being able to put out more watts per square metre is a big advantage,” says John Iannelli, president and founder of Caelux in Pasadena, California, which is developing tandems.
Some researchers and companies argue that perovskites might not need to match silicon’s 25-year warranties. Perovskites are improving so fast that if a module’s performance has declined over its first 15 years of life, it could simply be replaced with a better unit6. This repowering strategy is increasingly common for older silicon solar farms, at which the economic benefits of the more efficient technology outweigh the costs of removing and replacing ageing modules.
Political considerations are also driving investment in perovskites. In Europe, more renewable energy means less dependence on Russian gas supplies, and the United States is trying to reduce its reliance on Chinese PV supply chains, buoyed by spending and tax breaks from the 2022 Inflation Reduction Act. Some companies argue that the best way to achieve that is to avoid using silicon entirely. Several are developing all-perovskite tandems that contain two perovskite materials tuned to absorb different parts of the spectrum. “It means your supply chain is massively simplified,” says Tomas Leijtens, co-founder of Swift Solar in San Carlos, California, which is working on these silicon-free tandems.
Swift Solar is also part of a US academic–industry partnership called Tandems for Efficient and Advanced Modules using Ultrastable Perovskites (TEAMUP), which won $9 million of US Department of Energy (DOE) funding in April. Led by McGehee, its main aim is to develop perovskite–silicon tandem panels with an efficiency of greater than 28% and a performance degradation of less than 1% per year. Most of the firms working on perovskite cells in the United States are either in this partnership or in another project — which also won DOE funding this year. Led by Buonassisi at MIT, the project is called Accelerated Co-Design of Durable, Reproducible, and Efficient Perovskite Tandems (ADDEPT).
Still, many Chinese companies are also working on perovskite–silicon tandems and these might be poised to enter the market if the devices are successful. Shen says that LONGi has more than 100 people working on perovskite research and development, for example.
“I think it’d be very unwise to bet against China with solar manufacturing,” says Chase. The bottom line, she adds, is that the efficiency of solar panels is no longer a constraint on the global roll-out of solar power. Instead, the bottlenecks are the lack of electrical grid infrastructure for solar, and the high cost of batteries to store excess power. She argues that, for the next decade or so, it doesn’t matter whether perovskite tandems are successful or not — except to the companies involved. “I wish them luck, but solar will be enormous with or without perovskites,” she says.
Snaith, however, disagrees that improvements in silicon technology have narrowed the market space for cells incorporating perovskites. Any improvements in silicon PV also benefit tandems, making them more attractive than ever, he says. “We’re literally building on silicon. If silicon gets better, we get better.”