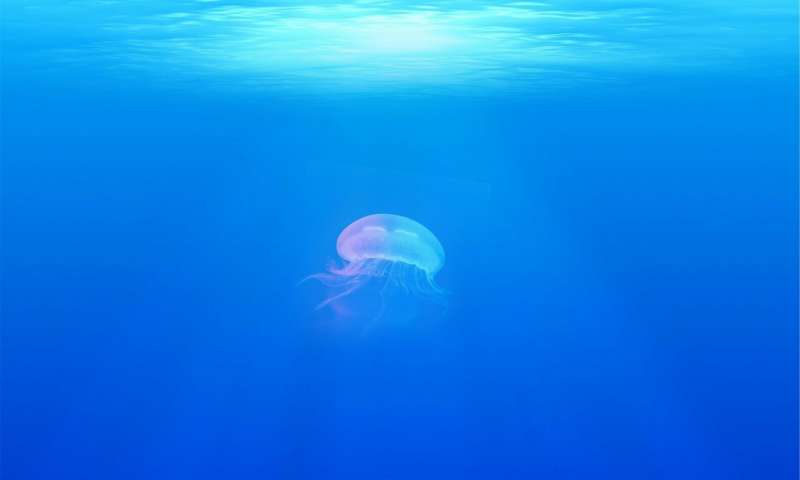
In the brain, when neurons fire off electrical signals to their neighbors, this happens through an “all-or-none” response. The signal only happens once conditions in the cell breach a certain threshold.
Now an MIT researcher has observed a similar phenomenon in a completely different system: Earth’s carbon cycle.
Daniel Rothman, professor of geophysics and co-director of the Lorenz Center in MIT’s Department of Earth, Atmospheric and Planetary Sciences, has found that when the rate at which carbon dioxide enters the oceans pushes past a certain threshold—whether as the result of a sudden burst or a slow, steady influx—the Earth may respond with a runaway cascade of chemical feedbacks, leading to extreme ocean acidification that dramatically amplifies the effects of the original trigger.
This global reflex causes huge changes in the amount of carbon contained in the Earth’s oceans, and geologists can see evidence of these changes in layers of sediments preserved over hundreds of millions of years.
Rothman looked through these geologic records and observed that over the last 540 million years, the ocean’s store of carbon changed abruptly, then recovered, dozens of times in a fashion similar to the abrupt nature of a neuron spike. This “excitation” of the carbon cycle occurred most dramatically near the time of four of the five great mass extinctions in Earth’s history.
Scientists have attributed various triggers to these events, and they have assumed that the changes in ocean carbon that followed were proportional to the initial trigger—for instance, the smaller the trigger, the smaller the environmental fallout.
But Rothman says that’s not the case. It didn’t matter what initially caused the events; for roughly half the disruptions in his database, once they were set in motion, the rate at which carbon increased was essentially the same. Their characteristic rate is likely a property of the carbon cycle itself—not the triggers, because different triggers would operate at different rates.
What does this all have to do with our modern-day climate? Today’s oceans are absorbing carbon about an order of magnitude faster than the worst case in the geologic record—the end-Permian extinction. But humans have only been pumping carbon dioxide into the atmosphere for hundreds of years, versus the tens of thousands of years or more that it took for volcanic eruptions or other disturbances to trigger the great environmental disruptions of the past. Might the modern increase of carbon be too brief to excite a major disruption?
According to Rothman, today we are “at the precipice of excitation,” and if it occurs, the resulting spike—as evidenced through ocean acidification, species die-offs, and more—is likely to be similar to past global catastrophes.
“Once we’re over the threshold, how we got there may not matter,” says Rothman, who is publishing his results this week in the Proceedings of the National Academy of Sciences. “Once you get over it, you’re dealing with how the Earth works, and it goes on its own ride.”
A carbon feedback
In 2017, Rothman made a dire prediction: By the end of this century, the planet is likely to reach a critical threshold, based on the rapid rate at which humans are adding carbon dioxide to the atmosphere. When we cross that threshold, we are likely to set in motion a freight train of consequences, potentially culminating in the Earth’s sixth mass extinction.
Rothman has since sought to better understand this prediction, and more generally, the way in which the carbon cycle responds once it’s pushed past a critical threshold. In the new paper, he has developed a simple mathematical model to represent the carbon cycle in the Earth’s upper ocean and how it might behave when this threshold is crossed.
Scientists know that when carbon dioxide from the atmosphere dissolves in seawater, it not only makes the oceans more acidic, but it also decreases the concentration of carbonate ions. When the carbonate ion concentration falls below a threshold, shells made of calcium carbonate dissolve. Organisms that make them fare poorly in such harsh conditions.
Shells, in addition to protecting marine life, provide a “ballast effect,” weighing organisms down and enabling them to sink to the ocean floor along with detrital organic carbon, effectively removing carbon dioxide from the upper ocean. But in a world of increasing carbon dioxide, fewer calcifying organisms should mean less carbon dioxide is removed.
“It’s a positive feedback,” Rothman says. “More carbon dioxide leads to more carbon dioxide. The question from a mathematical point of view is, is such a feedback enough to render the system unstable?”
“An inexorable rise”
Rothman captured this positive feedback in his new model, which comprises two differential equations that describe interactions between the various chemical constituents in the upper ocean. He then observed how the model responded as he pumped additional carbon dioxide into the system, at different rates and amounts.
He found that no matter the rate at which he added carbon dioxide to an already stable system, the carbon cycle in the upper ocean remained stable. In response to modest perturbations, the carbon cycle would go temporarily out of whack and experience a brief period of mild ocean acidification, but it would always return to its original state rather than oscillating into a new equilibrium.
When he introduced carbon dioxide at greater rates, he found that once the levels crossed a critical threshold, the carbon cycle reacted with a cascade of positive feedbacks that magnified the original trigger, causing the entire system to spike, in the form of severe ocean acidification. The system did, eventually, return to equilibrium, after tens of thousands of years in today’s oceans—an indication that, despite a violent reaction, the carbon cycle will resume its steady state.
This pattern matches the geological record, Rothman found. The characteristic rate exhibited by half his database results from excitations above, but near, the threshold. Environmental disruptions associated with mass extinction are outliers—they represent excitations well beyond the threshold. At least three of those cases may be related to sustained massive volcanism.
“When you go past a threshold, you get a free kick from the system responding by itself,” Rothman explains. “The system is on an inexorable rise. This is what excitability is, and how a neuron works too.”
Although carbon is entering the oceans today at an unprecedented rate, it is doing so over a geologically brief time. Rothman’s model predicts that the two effects cancel: Faster rates bring us closer to the threshold, but shorter durations move us away. Insofar as the threshold is concerned, the modern world is in roughly the same place it was during longer periods of massive volcanism.
In other words, if today’s human-induced emissions cross the threshold and continue beyond it, as Rothman predicts they soon will, the consequences may be just as severe as what the Earth experienced during its previous mass extinctions.
“It’s difficult to know how things will end up given what’s happening today,” Rothman says. “But we’re probably close to a critical threshold. Any spike would reach its maximum after about 10,000 years. Hopefully that would give us time to find a solution.”
“We already know that our CO2-emitting actions will have consequences for many millennia,” says Timothy Lenton, professor of climate change and earth systems science at the University of Exeter. “This study suggests those consequences could be much more dramatic than previously expected. If we push the Earth system too far, then it takes over and determines its own response—past that point there will be little we can do about it.”